Project
Rational Design of Therapeutics
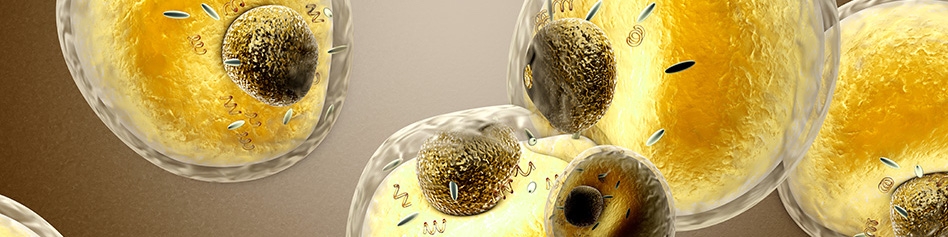
On-target mutations in infectious diseases often render inhibitors ineffective and are one of the key clinical failures of current therapies. We use HIV protease as a model system to understand mutation resistance. HIV protease substrates are unaffected or only moderately affected by resistance mutations that greatly decrease inhibitor binding. This idea has led to the design of broadly binding inhibitors using substrate mimicry. This is achieved by constraining inhibitors to bind within the consensus substrate volume, which we term the “substrate envelope”. However, while the substrate envelope has been relatively successful, some inhibitors that are designed based on this model are sensitive to mutants. We performed a detailed biophysical binding energy decomposition of a flat and susceptible binder pair and found that the susceptible inhibitor forms stronger interactions with key residues. These residues are entirely characterized by examining known resistant mutants to approved HIV protease inhibitors. To generalize our findings, we cross-validate on a set of ten HIV protease inhibitors with previously measured sensitivity. We find that interaction energy successfully classifies susceptible and flat inhibitors. Based on these results, we extend the current design paradigm. We develop a methodology to mitigate extraneous contacts with the active site and express it as an appropriate cost function, which is then minimized. We then implement this design scheme for HIV protease, yielding novel flat and susceptible binders. Put together, this work provides a framework for rational design of inhibitors for clinically-relevant targets.
Related Links
Contact us
If you would like to contact us about our work, please refer to our members below and reach out to one of the group leads directly.
Last updated Oct 13 '17